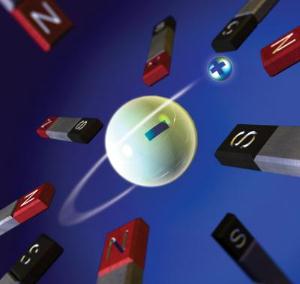
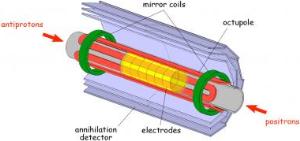
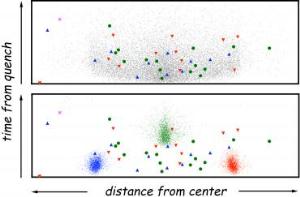
Atoms of antimatter have been trapped and stored for the first time by the ALPHA collaboration, an international team of scientists working at CERN, the European Organization for Nuclear Research near Geneva, Switzerland. Scientists from the U.S. Department of Energy's Lawrence Berkeley National Laboratory and the University of California at Berkeley have made key contributions to the ongoing international effort.
ALPHA stored atoms of antihydrogen, consisting of a single negatively charged antiproton orbited by a single positively charged anti-electron (positron). While the number of trapped anti-atoms is far too small to fuel the Starship Enterprise's matter-antimatter reactor, this advance brings closer the day when scientists will be able to make precision tests of the fundamental symmetries of nature. Measurements of anti-atoms may reveal how the physics of antimatter differs from that of the ordinary matter that dominates the world we know today.
Large quantities of antihydrogen atoms were first made at CERN eight years ago by two other teams. Although they made antimatter they couldn't store it, because the anti-atoms touched the ordinary-matter walls of the experiments within millionths of a second after forming and were instantly annihilated - completely destroyed by conversion to energy and other particles.
"Trapping antihydrogen proved to be much more difficult than creating antihydrogen," says ALPHA team member Joel Fajans, a scientist in Berkeley Lab's Accelerator and Fusion Research Division (AFRD) and a professor of physics at UC Berkeley. "ALPHA routinely makes thousands of antihydrogen atoms in a single second, but most are too 'hot'" - too energetic - "to be held in the trap. We have to be lucky to catch one."
The ALPHA collaboration succeeded by using a specially designed magnetic bottle called a Minimum Magnetic Field Trap. The main component is an octupole (eight-magnetic-pole) magnet whose fields keep anti-atoms away from the walls of the trap and thus prevent them from annihilating. Fajans and his colleagues in AFRD and at UC proposed, designed, and tested the octupole magnet, which was fabricated at Brookhaven. ALPHA team member Jonathan Wurtele of AFRD, also a professor of physics at UC Berkeley, led a team of Berkeley Lab staff members and visiting scientists who used computer simulations to verify the advantages of the octupole trap.
In the journal Nature ALPHA team reported the results of 335 experimental trials, each lasting one second, during which the anti-atoms were created and stored. The trials were repeated at intervals never shorter than 15 minutes. To form antihydrogen during these sessions, antiprotons were mixed with positrons inside the trap. As soon as the trap's magnet was "quenched," any trapped anti-atoms were released, and their subsequent annihilation was recorded by silicon detectors. In this way the researchers recorded 38 antihydrogen atoms, which had been held in the trap for almost two-tenths of a second.
"Proof that we trapped antihydrogen rests on establishing that our signal is not due to a background," says Fajans. While many more than 38 antihydrogen atoms are likely to have been captured during the 335 trials, the researchers were careful to confirm that each candidate event was in fact an anti-atom annihilation and was not the passage of a cosmic ray or, more difficult to rule out, the annihilation of a bare antiproton.
To discriminate among real events and background, the ALPHA team used computer simulations based on theoretical calculations to show how background events would be distributed in the detector versus how real antihydrogen annihilations would appear. Fajans and Francis Robicheaux of Auburn University contributed simulations of how mirror-trapped antiprotons (those confined by magnet coils around the ends of the octupole magnet) might mimic anti-atom annihilations, and how actual antihydrogen would behave in the trap.
Learning from antimatter
Before 1928, when anti-electrons were predicted on theoretical grounds by Paul Dirac, the existence of antimatter was unsuspected. In 1932 anti-electrons (positrons) were found in cosmic ray debris by Carl Anderson. The first antiprotons were deliberately created in 1955 at Berkeley Lab's Bevatron, the highest-energy particle accelerator of its day.
At first physicists saw no reason why antimatter and matter shouldn't behave symmetrically, that is, obey the laws of physics in the same way. But if so, equal amounts of each would have been made in the big bang - in which case they should have mutually annihilated, leaving nothing behind. And if somehow that fate were avoided, equal amounts of matter and antimatter should remain today, which is clearly not the case.
In the 1960s, physicists discovered subatomic particles that decayed in a way only possible if the symmetry known as charge conjugation and parity (CP) had been violated in the process. As a result, the researchers realized, antimatter must behave slightly differently from ordinary matter. Still, even though some antiparticles violate CP, antiparticles moving backward in time ought to obey the same laws of physics as do ordinary particles moving forward in time. CPT symmetry (T is for time) should not be violated.
One way to test this assumption would be to compare the energy levels of ordinary electrons orbiting an ordinary proton to the energy levels of positrons orbiting an antiproton, that is, compare the spectra of ordinary hydrogen and antihydrogen atoms. Testing CPT symmetry with antihydrogen atoms is a major goal of the ALPHA experiment.
How to make and store antihydrogen
To make antihydrogen, the accelerators that feed protons to the Large Hadron Collider (LHC) at CERN divert some of these to make antiprotons by slamming them into a metal target; the antiprotons that result are held in CERN's Antimatter Decelerator ring, which delivers bunches of antiprotons to ALPHA and another antimatter experiment.
Wurtele says, "It's hard to catch p-bars" - the symbol for antiproton is a small letter p with a bar over it - "because you have to cool them all the way down from a hundred million electron volts to fifty millionths of an electron volt."
In the ALPHA experiment the antiprotons are passed through a series of physical barriers, magnetic and electric fields, and clouds of cold electrons, to further cool them. Finally the low-energy antiprotons are introduced into ALPHA's trapping region.
Meanwhile low-energy positrons, originating from decays in a radioactive sodium source, are brought into the trap from the opposite end. Being charged particles, both positrons and antiprotons can be held in separate sections of the trap by a combination of electric and magnetic fields - a cloud of positrons in an "up well" in the center and the antiprotons in a "down well" toward the ends of the trap.
To join the positrons in their central well, the antiprotons must be carefully nudged by an oscillating electric field, which increases their velocity in a controlled way through a phenomenon called autoresonance.
"It's like pushing a kid on a playground swing," says Fajans, who credits his former graduate student Erik Gilson and Lazar Friedland, a professor at Hebrew University and visitor at Berkeley, with early development of the technique. "How high the swing goes doesn't have as much to do with how hard you push or how heavy the kid is or how the long the chains are, but instead with the timing of your pushes."
The novel autoresonance technique turned out to be essential for adding energy to antiprotons precisely, in order to form relatively low energy anti-atoms. The newly formed anti-atoms are neutral in charge, but because of their spin and the distribution of the opposite charges of their components, they have a magnetic moment; provided their energy is low enough, they can be captured in the octupole magnetic field and mirror fields of the Minimum Magnetic Field Trap.
Of the thousands of antihydrogen atoms made in each one-second mixing session, most are too energetic to be held and annihilate themselves against the trap walls.
Setting the ALPHA 38 free
After mixing and trapping - plus the "clearing" of the many bare antiprotons that have not formed antihydrogen - the superconducting magnet that produces the confining field is abruptly turned off - within a mere nine-thousandths of a second. This causes the magnet to "quench," a quick return to normal conductivity that results in fast heating and stress.
"Millisecond quenches are almost unheard of," Fajans says. "Deliberately turning off a superconducting magnet is usually done thousands of times more slowly, and not with a quench. We did a lot of experiments at Berkeley Lab to make sure the ALPHA magnet could survive multiple rapid quenches."
From the start of the quench the researchers allowed 30-thousandths of a second for any trapped antihydrogen to escape the trap, as well as any bare antiprotons that might still be in the trap. Cosmic rays might also wander through the experiment during this interval. By using electric fields to sweep the trap of charged particles or steer them to one end of the detectors or the other, and by comparing the real data with computer simulations of candidate antihydrogen annihilations and look-alike events, the researchers were able to unambiguously identify 38 antihydrogen atoms that had survived in the trap for at least 172 milliseconds - almost two-tenths of a second.
Says Fajans, "Our report in Nature describes ALPHA's first successes at trapping antihydrogen atoms, but we're constantly improving the number and length of time we can hold onto them. We're getting close to the point where we can do some classes of experiments on antimatter atoms. The first attempts will be crude, but no one has ever done anything like them before."
University of Liverpool - Press Release:
Scientists step closer to understanding secrets of anti-matter
Researchers have trapped and held the atoms, the anti-matter counterpart of hydrogen, using an experiment called ALPHA at CERN in Switzerland. Anti-matter particles are instantly annihilated when they come into contact with matter and, until now, it has not been possible to study anti-hydrogen in any detail. The experiment has brought scientists closer to understanding the structure and composition of anti-matter.
The ALPHA experiment was developed to cool and slow down the anti-particles that make up anti-hydrogen and mix them to produce antihydrogen. Some of the anti-atoms are then trapped by electromagnets inside the machine, giving researchers the time to study them. Liverpool scientists constructed the silicon detector system at the heart of the experiment to identify when anti-hydrogen is present inside the experiment.
Professor Paul Nolan, from the University of Liverpool's Department of Physics, explains: "When antihydrogen decays inside the ALPHA experiment, it emits particles, called pions, from the point at which it exists. Our detector surrounds the area where antihydrogen is formed and for each pion emitted we get three points as it travels outwards. Using a computer we can then construct a line between these points and trace it back to the origin of the antihydrogen. Being able to study these particles brings us closer to understanding the composition of anti-matter and the physical properties of our Universe."
Particles that are known to exist in the Universe have their mirror image in anti-matter, but with the opposite electrical charge. It is thought that when the Universe formed, matter and anti-matter existed in equal measure, until they collided and annihilated, with the residual matter making up the planets of the solar system. Although anti-matter is known to exist - in the stars for example - scientists have questioned for many year why it is only matter that can be seen in our physical world today.
Professor Mike Charlton, at Swansea University, added: "Hydrogen is the simplest of all atoms, and antihydrogen is the easiest type of anti-matter to produce in the laboratory. Understanding it will hopefully enable us to shed light on why almost everything in the known Universe consists of matter, rather than anti-matter."
University of Calgary - Press Release:
Researchers trap antimatter atoms - discovery may challenge physics fundamentals
In the movie Angels and Demons, scientists have solved one of the most perplexing scientific problems: the capture and storage of antimatter. In real life, trapping atomic antimatter has never been accomplished, until now.
A team made up of researchers from the University of Calgary, institutions across Canada and around the world have discovered how to trap atomic antimatter.
"This is a major discovery. It could enable experiments that result in dramatic changes to the current view of fundamental physics or in confirmation of what we already know now," says Rob Thompson, head of physics and astronomy at the University of Calgary and co-investigator in the ALPHA collaboration, one of two teams competing to gain a better understanding of antimatter and our universe.
Both teams, ALPHA and the Harvard-led ATRAP, have been at this race for over five years conducting experiments in close quarters at CERN (European Organization for Nuclear Research), the world's largest particle physics lab, located in the suburbs of Geneva, Switzerland. CERN is the only laboratory in the world with the proper equipment where this research can be carried out.
"These are significant steps in antimatter research," said CERN Director General Rolf Heuer, "and an important part of the very broad research programme at CERN."
The goal of the competition involves trapping and storing the simplest of all antimatter atoms, antihydrogen, with the purpose of studying it. Hydrogen is the lightest and most abundant chemical element.
"We know a lot about matter, but very little about antimatter. We assume there was as much antimatter created in the Big Bang as matter. There are many questions. Where is the antimatter? Where did it go? And why does it appear that there is more matter than antimatter?" says Makoto Fujiwara, adjunct professor in the Department of Physics and Astronomy at the University of Calgary and a research scientist at TRIUMF, Canada's national laboratory for particle and nuclear physics.
ALPHA-Canada scientists and students have been playing leading roles in the experiment. "It's been a rare privilege and a tremendous learning experience taking part in this groundbreaking international endeavour," says Richard Hydomako, a PhD student at the University of Calgary.
Trapping antimatter is tricky. When matter and antimatter get too close, they destroy each other, in a kind of explosion, leaving behind the energy which made them. The challenge is cooling the atoms off enough, 272 degrees below zero, so that they are slow enough to be trapped in a magnetic storage device.
"We've been able to trap about 38 atoms, which is an incredibly small amount, nothing like what we would need to power Star Trek's starship Enterprise or even to heat a cup of coffee," says Thompson , one of 42 co-authors of the Nature paper along with the University of Calgary's Makoto Fujiwara and graduate students Richard Hydomako and Tim Friesen.
"Now we can start working on the next step which is to use tools to study it," adds Thompson.
University of California - Press Release:
Antihydrogen trapped for first time
CERN antiprotons combined with positrons at low speed and captured in magnetic trap.
Physicists working at the European Organization for Nuclear Research (CERN) in Geneva, Switzerland, have succeeded in trapping antihydrogen - the antimatter equivalent of the hydrogen atom - a milestone that could soon lead to experiments on a form of matter that disappeared mysteriously shortly after the birth of the universe 14 billion years ago.
The first artificially produced low energy antihydrogen atoms - consisting of a positron, or antimatter electron, orbiting an antiproton nucleus - were created at CERN in 2002, but until now the atoms have struck normal matter and annihilated in a flash of gamma-rays within microseconds of creation.
The ALPHA (Antihydrogen Laser PHysics Apparatus) experiment, an international collaboration that includes physicists from the University of California, Berkeley, and Lawrence Berkeley National Laboratory (LBNL), has now trapped 38 antihydrogen atoms, each for more than one-tenth of a second.
While the number and lifetime are insufficient to threaten the Vatican - in the 2000 novel and 2009 movie "Angels and Demons," a hidden vat of potentially explosive antihydrogen was buried under St. Peter's Basilica in Rome - it is a starting point for learning new physics, the researchers said.
"We are getting close to the point at which we can do some classes of experiments on the properties of antihydrogen," said Joel Fajans, UC Berkeley professor of physics, LBNL faculty scientist and ALPHA team member. "Initially, these will be crude experiments to test CPT symmetry, but since no one has been able to make these types of measurements on antimatter atoms at all, it's a good start."
CPT (charge-parity-time) symmetry is the hypothesis that physical interactions look the same if you flip the charge of all particles, change their parity - that is, invert their coordinates in space - and reverse time. Any differences between antihydrogen and hydrogen, such as differences in their atomic spectrum, automatically violate CPT, overthrow today's "standard model" of particles and their interactions, and may explain why antimatter, created in equal amounts during the universe's birth, is largely absent today.
Antimatter, first predicted by physicist Paul Dirac in 1931, has the opposite charge of normal matter and annihilates completely in a flash of energy upon interaction with normal matter. While astronomers see no evidence of significant antimatter annihilation in space, antimatter is produced during high-energy particle interactions on earth and in some decays of radioactive elements. UC Berkeley physicists Emilio Segre and Owen Chamberlain created antiprotons in the Bevatron accelerator at the Lawrence Radiation Laboratory, now LBNL, in 1955, confirming their existence and earning the scientists the 1959 Nobel Prize in physics.
Slow antihydrogen was produced at CERN in 2002 thanks to an antiproton decelerator that slowed antiprotons enough for them to be used in experiments that combined them with a cloud of positrons. The ATHENA experiment, a broad international collaboration, reported the first detection of cold antihydrogen, with the rival ATRAP experiment close behind.
The ATHENA experiment closed down in 2004, to be superseded by ALPHA, coordinated by Jeffrey Hangst of the University of Aarhus in Denmark. Since then, the ALPHA and ATRAP teams have competed to trap antihydrogen for experiments, in particular, laser experiments to measure the antihydrogen spectrum (the color with which it glows) - and gravity measurements. Before the recent results, the CERN experiments have produced - only fleetingly - tens of millions of antihydrogen atoms, Fajans said.
ALPHA's approach was to cool antiprotons and compress them into a matchstick-size cloud (20 millimeters long and 1.4 millimeters in diameter). Then, using autoresonance, a technique developed by UC Berkeley visiting professor Lazar Friedland and first explored in plasmas by Fajans and former U.C Berkeley graduate student Erik Gilson, the cloud of cold, compressed antiprotons is nudged to overlap a like-size positron cloud, where the two particles mate to form antihydrogen.
All this happens inside a magnetic bottle that traps the antihydrogen atoms. The magnetic trap is a specially configured magnetic field that Fajans and then-UC Berkeley undergraduate Andrea Schmidt first proposed, using an unusual and expensive octupole superconducting magnet to create a more stable plasma.
"For the moment, we keep antihydrogen atoms around for at least 172 milliseconds - about a sixth of a second - long enough to make sure we have trapped them," said colleague Jonathan Wurtele, UC Berkeley professor of physics and LBNL faculty scientist. Wurtele collaborated with LBNL visitor Katia Gomberoff, staff members Alex Friedman, David Grote and Jean-Luc Vay and with Fajans to simulate the new and original magnetic configurations.
Trapping antihydrogen isn't easy, Fajans said, because it is a neutral, or chargeless, particle. Magnetic bottles are generally used to trap charged particles, such as ionized atoms. These charged particles spiral along magnetic field lines until they encounter an electric field that bounces them back towards the center of the bottle.
Neutral antihydrogen, however, would normally be unaffected by these fields. But the team takes advantage of the tiny magnetic moment of the antihydrogen atom to trap it using a steeply increasing field - a so-called magnetic mirror - that reflects them backward toward the center. Because the magnetic moment is so small, the antihydrogen has to be very cold: less than about one-half degree above absolute zero (0.5 Kelvin). That means the team had to slow down the antiprotons by a factor of one hundred billion from their initial energy emerging from the antiproton decelerator.
Once trapped, the experimenters sweep out the lingering antiprotons with an electric field, then shut off the mirror fields and let the trapped antihydrogen atoms annihilate with normal matter. Surrounding detectors are sensitive to the charged pions that result from the proton-antiproton annihilation. Cosmic rays can also set off the detector, but their straight-line tracks can be easily distinguished, Fajans said. A few antiprotons could potentially remain in the trap, and their annihilations would look similar to those of antihydrogen, but the physicists' simulations show that such events can also be successfully distinguished from antihydrogen annihilations.
During August and September of 2010, the team detected an antihydrogen atom in 38 of the 335 cycles of antiproton injection. Given that their detector efficiency is about 50 percent, the team calculated that it captured approximately 80 of the several million antihydrogen atoms produced during these cycles. Experiments in 2009 turned up six candidate antihydrogen atoms, but they have not been confirmed.
ALPHA continues to detect antihydrogen atoms at an increasing rate as the experimenters learn how to better tune their experiment, Fajans said.
Further Information:
G. B. Andresen et al.:
Trapped antihydrogen.
In: Nature; advance online publication 17 November 2010, DOI 10.1038/nature09610
Eugenie Samuel Reich:
Antimatter held for questioning. Magnetically trapped atoms could test fundamental physics.
In: Nature; 468, 355, 17 November 2010, DOI 10.1038/468355a
Quelle: DOE/Lawrence Berkeley National Laboratory. Berkeley Lab is a U.S. Department of Energy national laboratory managed by the University of California for the DOE Office of Science.
Last update: 17.11.2010
Perma link: https://www.internetchemistry.com/news/2010/nov10/antihydrogen-atom-trapped.php
More chemistry: index | chemicals | lab equipment | job vacancies | sitemap
Internetchemistry: home | about | contact | imprint | privacy
© 1996 - 2023 Internetchemistry